Lessons in Antisense
Lesson 1 – Introduction
April 14, 2025 by Dr. Stan Crooke
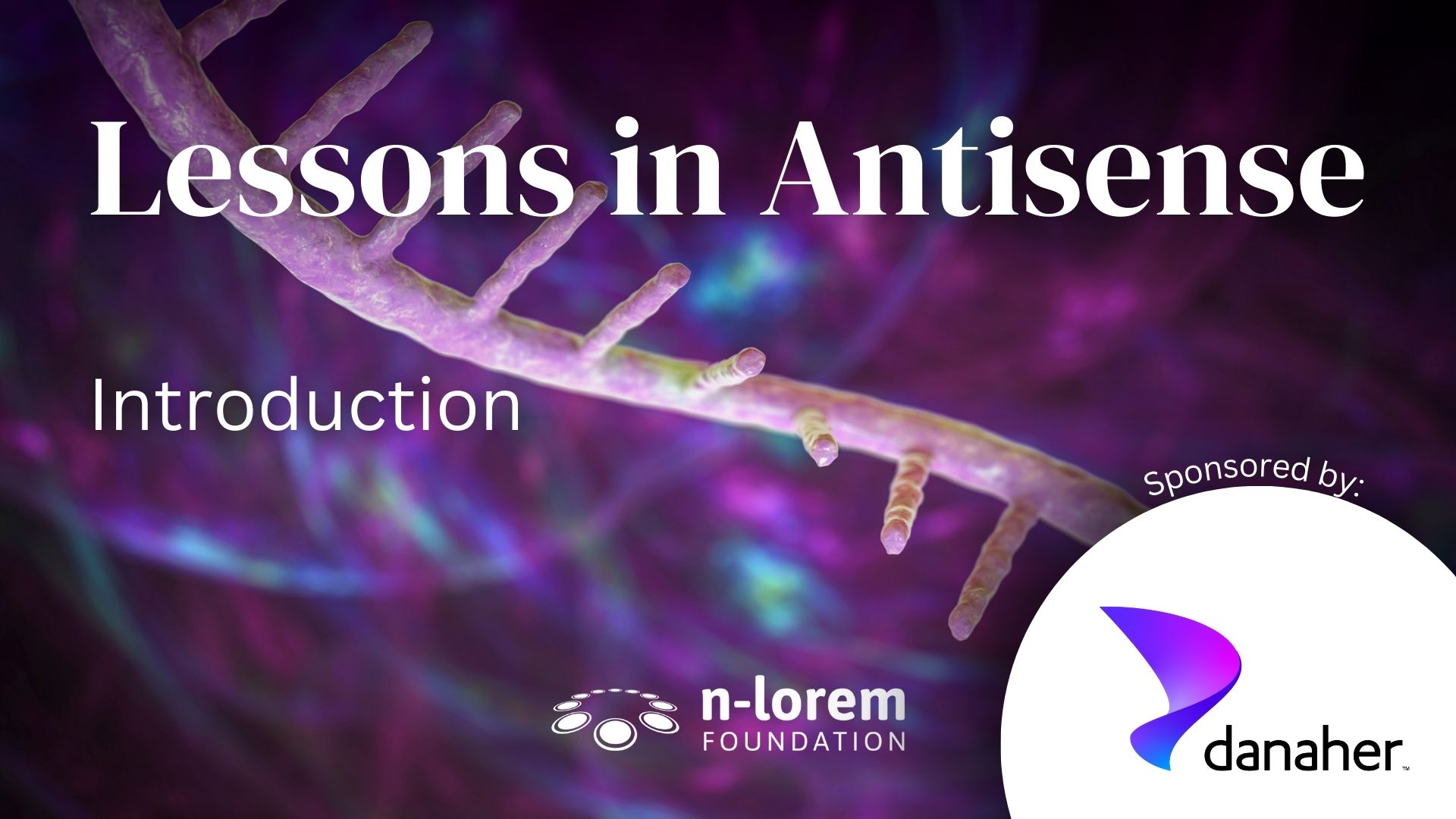
Introduction
RNA-targeted drug discovery (RTD) is poised to take its place in the mainstream of medicine as a new, more efficient, broadly enabling drug discovery platform and is particularly advantageous for genetically caused diseases. Though RNA-targeted drug discovery is “the same old dance” of traditional drug discovery, the dance steps are different enough that even solid scientists experienced in traditional drug discovery must undergo a steep learning curve. In my experience, on average, it takes experienced, bright, well-trained scientists two years or so to become familiar enough with the basic principles and characteristics of the technology to make the type of informed judgements required to match RTD to molecular targets and therapeutic opportunities in a consistently effective manner.
To shorten the time required to become an expert in RNA-targeted drug technology for drug discovery, I put together what I think of as Cliff Notes focused on antisense technology as an effort to facilitate learning at n-Lorem. Given the broad interest in antisense, I have formalized these lessons a bit and am making them available for anyone interested.
Obviously, the lessons are abbreviations of conclusions of more than 35 years of scientific effort. So, by definition, they are superficial, and they reflect my biases and limitations. For example, I am not a chemist or biophysicist, but several of the lessons must focus on areas like these in which I am not formally trained.
One more caveat to keep in mind: The technology continues to evolve rapidly, and as we better understand basic principles, they may change, as may our perceptions about the strengths and limitations of the technology. Nevertheless, I hope that you find these lessons of some use.
Basic Principles
RTD technology is a chemically-based synthetic drug discovery platform. Consequently, the single most critical step in the creation of RTD technology was the invention of the medicinal chemistry of oligonucleotides, a discipline that did not exist in 1989 when I founded Ionis Pharmaceuticals. All medicinal chemistry seeks to modify chemicals to enhance the ability of chemicals to perform certain tasks that we think of as therapeutic goals. The properties of all drugs can be divided into pharmacological (the desired effects), pharmacokinetic (how the drug is absorbed, distributed, and cleared, and the times taken for each of those steps) and toxicological (undesired effects). Similarly, the medicinal chemistry of oligonucleotides sought to instill good drug properties into oligonucleotides.
Dan Cook and I created the medicinal chemistry plan, which he implemented at Ionis. Though we decided to modify all sites other than those required for Watson-Crick hydrogen bonding; from the beginning, the primary foci were on the 2’-position of the sugar in a nucleotide and replacing the phosphate as the inter-nucleotide linkage. The 2’-position in ribose was known to determine the shape of the ribose and its position relative to the nucleobase, and these characteristics defined the affinity for a target RNA. Additionally, the 2’-OH in the ribose was known to be critical for nuclease cleavage of an oligonucleotide. So, we reasoned that we might enhance potency if we modified oligonucleotides with the right 2’-position and create oligonucleotides that had a longer duration of action because they would be relatively resistant to degradation by nucleases.
Of course, it was obvious that the phosphate as the inter-nucleotide linkage would have to be replaced to avoid rapid nuclease digestion, but it wasn’t obvious what type of phosphate replacement would be best. The choices were neutral—methyl-phosphonates or morpholinos—or charged—phosphorothioates. I reasoned that a neutral phosphate replacement and the lipophilic nucleobases would result in molecules that would not distribute well but be trapped in lipid depots or membranes while phosphorothioate (PS) linkages would create an amphipathic oligonucleotide that would have a better chance of distributing broadly in the body and in cells. Both opinions turned out to be correct, but PS linkages proved vital for reasons entirely different from what I thought in 1989.
General Principles
The single most important step in creating the technology was the establishment of the medicinal chemistry of oligonucleotides.
As with all drug discovery, the medicinal chemistry of oligonucleotides seeks to enhance the pharmacological (PD), pharmacokinetic (PK), and toxicological (TD) properties of oligonucleotides.
Two forms of RTDs are used today, single (antisense oligonucleotides or ASOs) and double stranded oligonucleotides (small interfering RNAs or siRNAs). They differ chemically and those differences result in distinct pharmacological, pharmacokinetic, and toxicological profiles.
The Medicinal Chemistry of Oligonucleotides
PS DNA or RNA has inadequate RNA affinities to be active at safe doses in humans
Conclusions
Thanks to more than three decades of research, a great deal is understood about the behavior of PS ASOs and the molecular mechanisms responsible for their behavior. The key point is that PS ASOs of various chemical classes are complex chemicals that interact not only with RNA, but with many proteins. The interactions with proteins are affected by PS content, the nature of 2’-modifications and sequence.
Because PS ASOs are amphipathic, relatively small and flexible, and bind to plasma proteins, they distribute broadly to peripheral tissues. At low doses, the organs that are well vascularized accumulate the most PS ASO, but at higher doses, the primary tissues saturate, and PS ASOs can be delivered to secondary organs. PS ASOs metabolized by nucleases into fragments that no longer bind effectively to proteins (because they have too few PS) and are cleared from cells and organs and filtered by the kidney into urine.
Distribution from plasma to peripheral organs is rapid (t1/2 distribution: about 1 hour) and cleared slowly from tissues, facilitating infrequent doses. We also have solid understanding of sub-organ PK/PD properties and use that knowledge to guide target selection and dosing.
Cell uptake and sub-cellular distribution are affected by protein binding and most productive delivery of PS ASOs occurs via endosomal processes.
Targeted delivery to some specific organs and cells has been accomplished, but skeletal muscle, cardiac muscle, some immune cells, and most solid cancers remain challenging sites to which one can deliver PS ASOs.
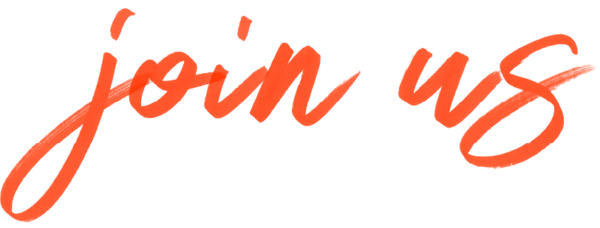
We cannot do
this alone
Together we are changing the world—
one patient at a time
We hope that you join us on this journey to discover, develop and provide individualized antisense medicines for free for life for nano-rare patients. The ultimate personalized medicine approach – for free, for life.
Follow us on social for updates on our latest efforts